Narrow Linewidth Lasers
The frequency (or color) of the electromagnetic field generated by a laser is usually a well-defined tone. A useful way to quantify the "cleanliness" of that tone, is by using a quantity called linewidth. The narrower the linewidth is, the cleaner the laser frequency is.
The mechanism that limits how narrow that line is, can be described in general as "noise". Fluctuations inside the laser's cavity contribute to the broadening of that line. For example, a well-known source of noise is called spontaneous emission, and is related to quantum fluctuations of the vacuum. Other sources of fluctuations, such as random changes of electron densities in the cavity, temperature fluctuations and others also contribute to the noise.
A laser with a spectrum that is very well behaved and defined is categorized as a narrow-linewidth-laser. Narrow-linewidth lasers play an important role in a wide variety of applications, from sensing and spectroscopy to optical communication and on-chip clocks.
Current narrow-linewidth systems are usually implemented in doped fibers and are big, expensive and power-hungry. On the other hand, semiconductor lasers are superior in size, cost and power consumption, but their linewidth is historically limited to the sub-MHz regime.
The main source of noise that limits the linewidth of most commercial semiconductor lasers is spontaneous emission. This is a quantum mechanical phenomena where excited electrons 'spontaneously" decay while emitting a photon into that laser mode. That photon is in random phase compare to the laser field, and therefore "jitters" its phase, and introduces noise. This is illustrated in the phasor diagram below:
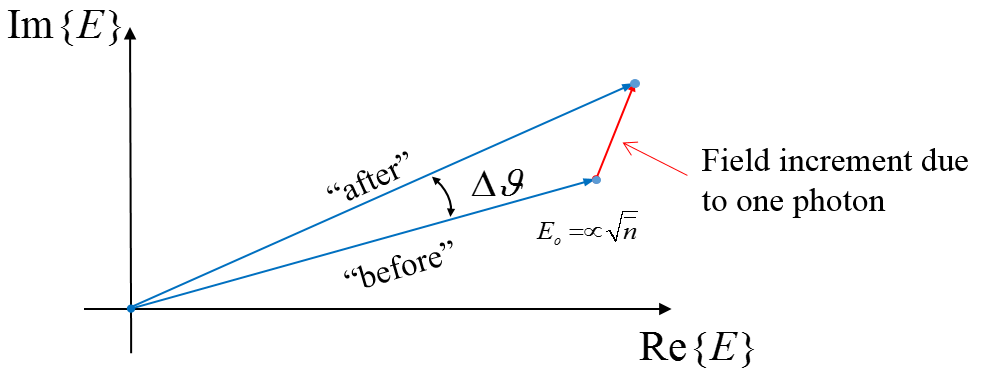
The same excited electrons are also providing gain, or amplification, to the laser field. For that reason, laser designers historically attempted to confine these 'gain' electrons as close as possible to the laser's optical mode. The common consensus was that this approach makes the most efficient lasers.
It turns out that this approach is also what limited the linewidth of semiconductor lasers for generations. Electrically-conductive materials are also optically 'lossy'. When the laser cavity has a lot of loss, its "quality factor" is poor. In other words, light cannot be efficiently stored in a lossy resonator.
Not only does channels of loss are also channels of noise (this is a fundamental result manifested in the fluctuations-dissipation theorem), but also each spontaneous emission event has a bigger effect on the overall phase, simply since there aren't so many photons in the cavity.
Putting the 'gain' electrons far away from the optical mode will indeed yield less spontaneous emission into the laser mode, but their ability to provide optical gain will be reduced by the same amount. However, and this is key - a laser only needs enough gain to overcome loss.
Lets assume we have a high quality resonator, and we attach gain electrons directly on it. These electrons will provide gain, but also loss, and the overall cavity will no longer be of high quality. Now assume we start to move these electrons away from the optical mode. The optical gain will of course decrease. However, because losses went down as well, we don't need that much modal gain anyway…
Continuing this line thought, we will end up with narrower linewidth lasers, with no sacrifice in power consumption, or threshold current. We just need to have a high quality resonator, and make sure the gain electrons are not too close. Just close enough to provide enough gain to overcome the losses.
The figure below shows a generic narrow-linewdith design:
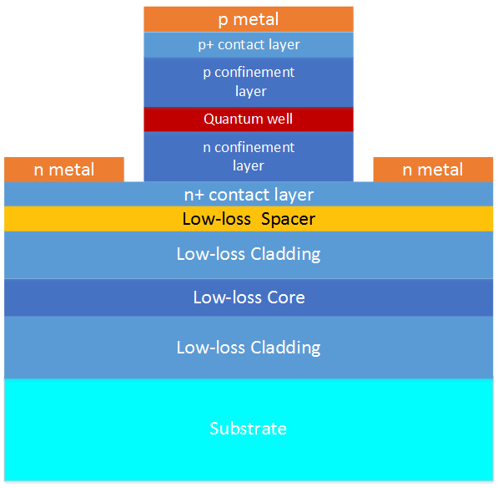
A key feature in this design, is that the current does not flow through the optical mode (conductive materials are lossy). Another important feature, is the physical separation between the low-loss regions, and the lossy gain medium.
We implemented that design on the hybrid Si/III-V platform. We fabricated high quality Si resonators (Q > 1 million!), and attached III-V gain medium, such that the overall quality factors are still high, and we have just enough gain to overcome losses. We have demonstrated semiconductor lasers with record-breaking sub-KHz linewidth. This is roughly three orders of magnitude better than traditional semiconductor lasers!
The figure below shows the experimental frequency noise spectrum of lasers of different designs. It demonstrates how modal control is used to bring the laser's linewidth from 40 KHz (already excellent result) down to 1 KHz.
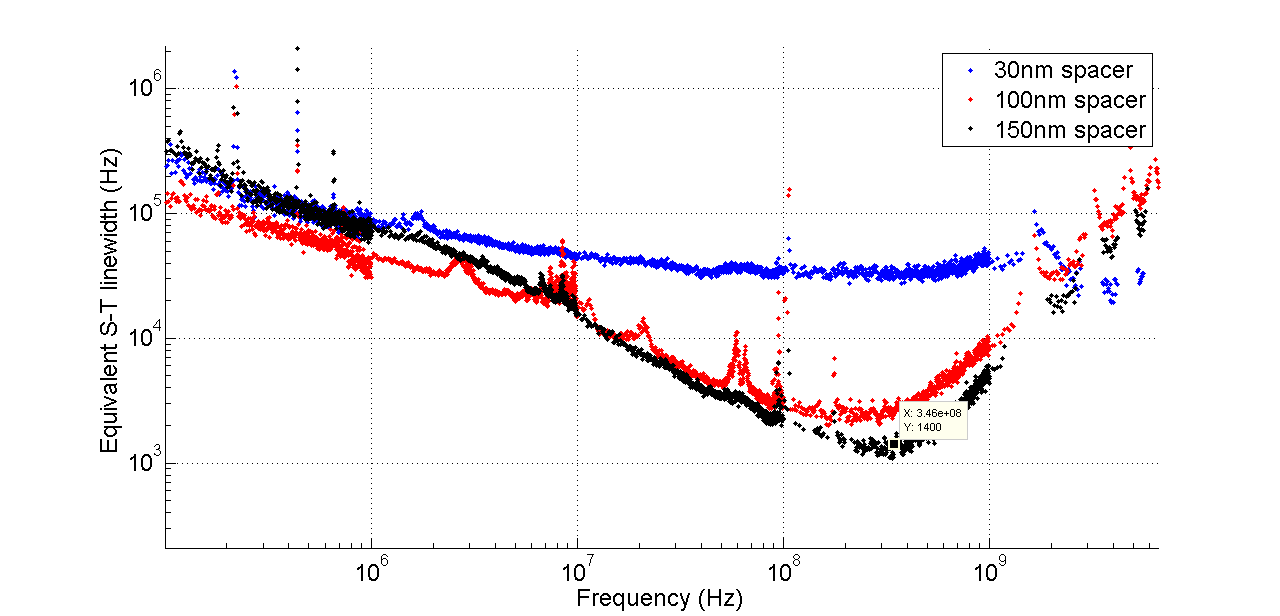
Want to learn more? See our paper in the proceeding of the national academy of science.